Drug-free macromolecular therapeutics (DFMT) is a B cell depletion strategy thet mimics the mechanism of immune effector cells to crosslink surface receptors of target cells and induce apoptosis. The receptor crosslinking is mediated by the biorecognition of high-fidelity natural binding motifs (antibody fragments, coiled-coil peptides, or complementary oligonucleotides) that are grafted to the side chains of synthetic polymers. The designed therapeutics have been tested against B-cell malignancies that highly express the surface antigen CD20. In these designs, two hybrid nanoconjugates are being used: (1) an anti-CD20 Fab’ antibody fragment attached to an oligonucleotide1 (or peptide1); (2) a linear N-(2-hydroxypropyl)methacrylamide (HPMA) copolymer or human serum albumin (HSA) grafted with multiple copies of complementary oligonucleotide2 (or peptide2). The conjugate pairs self-assemble via oligonucleotide hybridization (or coiled-coil peptide formation) at the surface of CD20 B-cells, which crosslinks CD20 antigens and initiates apoptosis (Figure 1).
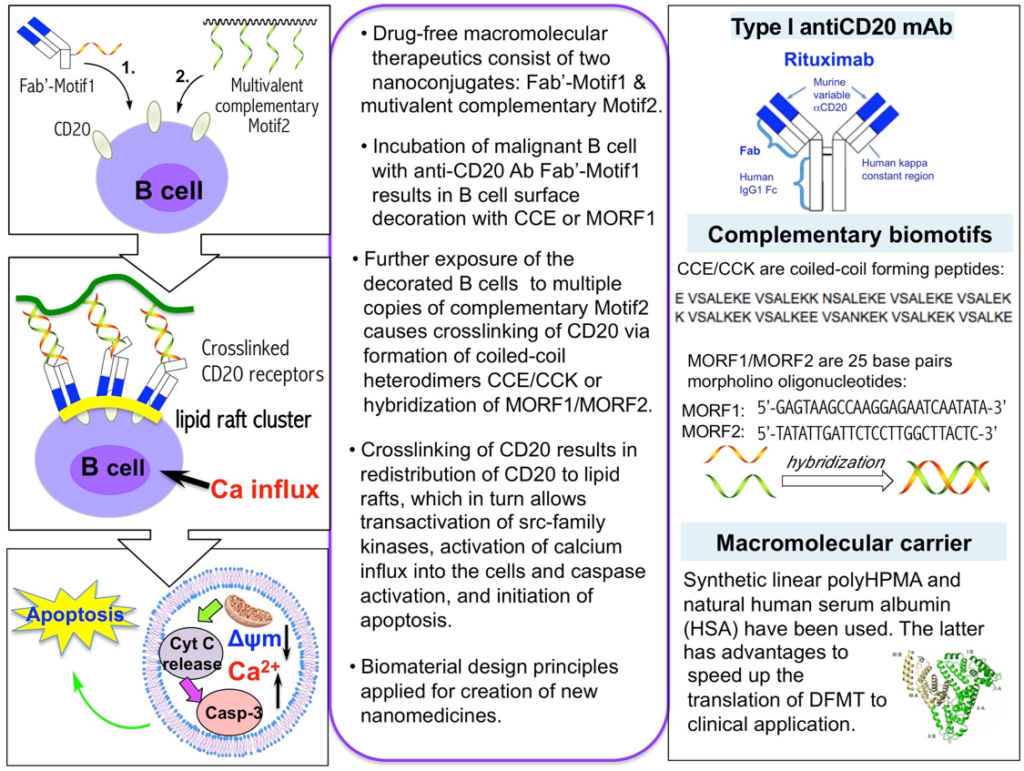
This new paradigm in macromolecular therapeutics is efficient in vitro [1], in vivo [3-7], and on cells from patients diagnosed with various blood borne cancers [8]. As a B cell depletion strategy, this approach was effective in a collagen-induced rheumatoid arthritis mouse model [9].
References
[1] Drug-Free Macromolecular Therapeutics: Induction of Apoptosis by Coiled-Coil-Mediated Cross-Linking of Antigens on the Cell Surface. K Wu, J Liu, R N Johnson, J Yang, J Kopeček. Angew. Chem. Int. Ed.. 122 (2010): 1493-1497. [doi] [2] Cell surface self-assembly of hybrid nanoconjugates via oligonucleotide hybridization induces apoptosis. T-W Chu, J Yang, R Zhang, M Sima, J Kopeček. ACS Nano. 8 (2014): 719-730. [doi] [3] Drug-free macromolecular therapeutics – a new paradigm in polymeric nanomedicines. T-W Chu, J Kopeček. Biomaterials Sci.. 3 (2015): 908-922. [doi] [4] Super-Resolution Imaging and Quantitative Analysis of Membrane Protein/Lipid Raft Clustering Mediated by Cell-Surface Self-Assembly of Hybrid Nanoconjugates. J M Hartley, T-W Chu, E M Peterson, R Zhang, J Yang, J Harris, J Kopeček. ChemBioChem. 16 (2015): 1725-1729. [doi] [5] Human Serum Albumin Based Drug-Free Macromolecular Therapeutics: Apoptosis Induction by Coiled-Coil-Mediated Cross-Linking of CD20 Antigens on Lymphoma B Cell Surface. L. Zhang, Y. Fang, L. Li, J. Yang, D.C. Radford, J. Kopeček. Macromol. Biosci. 18 (2018): 1800224; [doi] [6] Drug-Free Albumin-Triggered Sensitization of Cancer Cells to Anticancer Drugs. L. Li, J. Yang, S. Soodvilai, J. Wang, P. Opanasopit, J. Kopeček. J. Controlled Release 293 (2019) 84-93. [doi] [7] Biorecognition: A Key to Drug-free Macromolecular Therapeutics. J. Yang, L. Li, J. Kopeček. Biomaterials 190-191 (2019): 11-23. [doi] [8] Drug-free Macromolecular Therapeutics Induce Apoptosis in Cells Isolated from Patients with B Cell Malignancies with Enhanced Apoptosis Induction by Pretreatment with Gemcitabine. J. Wang, L. Li, J. Yang, P.M. Clair, M. Glenn, D.M. Stephens, D.C. Radford, K.M. Kosak, M.W. Deininger, P.J. Shami, J. Kopeček. Nanomedicine: NBM 16 (2019): 217-225. [doi] [9] Exploration and Evaluation of Therapeutic Efficacy of Drug-Free Macromolecular Therapeutics in Collagen-Induced Rheumatoid Arthritis Mouse Model. J. Wang, Y. Li, L. Li, J. Yang, J. Kopeček. Macromol. Biosci. (2020) 1900445. [doi] [10] Amplification of CD20 Crosslinking in Rituximab Resistant B-Lymphoma Cells Enhances Apoptosis Induction by Drug-Free Macromolecular Therapeutics. L. Li, J. Yang, J. Wang, J. Kopeček, ACS Nano 12, 3658-367. [doi] [11] Nanomedicines in B Cell-Targeting Therapies. J. Wang, J. Yang, J. Kopeček, Acta Biomater. 137, 1-19 (2022). [doi]2nd generation of DMFT
Our present studies evaluate the 2nd generation of DMFT. Anti-CD20 antibodiesare divided into Type I such as rituximab (RTX) and Type II such as obinutuzumab (OBN); they have different patterns of binding to CD20 receptor. RTX binds between CD20 tetramers resulting in accumulation in lipid rafts, calcium influx and caspase activation. OBN binds within one tetramer with the conformation compatible with homotypic adhesion regions, leading to actin cytoskeleton remodeling and lysosome disruption. Our design enhances the activity of Type II OBN by triggering the apoptosis activation pathways of both types of antibodies (Figure 2). This new system is composed of two nanoconjugates: a) bispecific engager, OBN-MORF1 (OBN conjugated to one morpholino oligonucleotide MORF1); and b) a crosslinking (effector) component HSA-(MORF2)X (human serum albumin (HSA) grafted with multiple copies of complementary morpholino oligonucleotide 2). Modification of OBN with one MORF1 does not impact the binding of OBN-MORF1 to CD20 and following binding to CD20 Type II effects occur. Further exposure to multivalent effector HSA-(MORF2)X results in clustering the OBN-MORF1-CD20 complexes into lipid rafts and Type I effects occur. This new approach, called “clustered OBN (cOBN)” combines effects of both antibody types resulting in very high apoptotic levels [10].
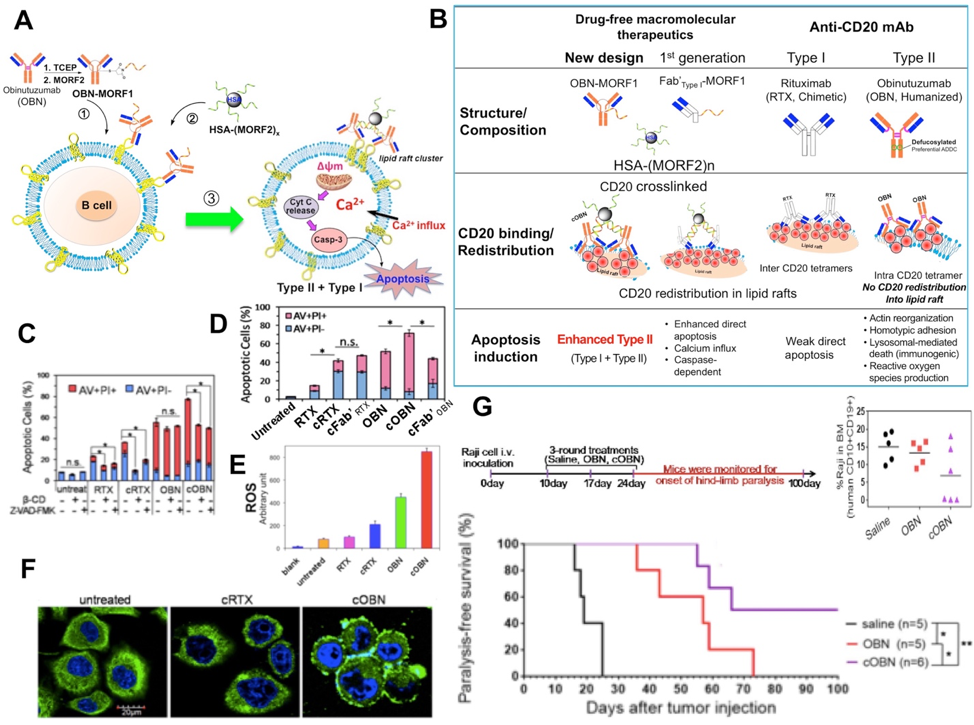